
The Karpac Lab is broadly interested in the origins of signaling networks that provide animals with metabolic flexibility, and thus the capacity to balance energy homeostasis. These ancient networks, under intense evolutionary pressure, both respond to and are shaped by diverse inputs, such as nutrient availability, pathogens, and aging. We primarily use the fruit fly Drosophila melanogaster as a genetic model to investigate the function and integration of these signaling networks at multiple levels of biological organization: from molecules, to cells and tissues, to inter-organ communication, to organismal physiology and aging. Through collaborations, we are also exploiting unique features of our Drosophila models, in combination with mouse and human models, to explore disease signaling mechanisms. Below are some examples of the questions we are currently exploring in the lab (as well as our funding sources):

CELLULAR AND SYSTEMIC COORDINATION OF ENERGY HOMEOSTASIS
for example: How do diverse tissues with unique metabolic functions coordinate signaling pathway responses to autonomously and systemically balance energy homeostasis?
How does the spatial organization of metabolic organs (i.e. proximity to other metabolic organs) within a complex body plan influence the balance of energy homeostasis?
How does nutrient availability or diet shape the complexity of metabolic signaling pathway integration (both acutely and evolutionarily)?
Animals must carefully balance energy use with energy demands, primarily through the control of carbohydrate, lipid, and amino acid metabolism, in order to promote complex biological process such as development, growth, and reproduction. This balance is also required to drive metabolic adaption during shifts from food abundance to food scarcity, or in response to more acute changes in dietary composition and feeding behaviors. Furthermore, the evolution of complex organ systems in metazoans has dictated that the maintenance of energy homeostasis requires coordinating local and systemic energy demands between organs with specialized functions. Fruit flies, like many animals, store large amounts of lipids for energy in functionally analogous tissues, using evolutionarly conserved enzymatic and metabolic signaling pathways regulating lipid breakdown, synthesis, and usage. Drosophila is thus emerging as an important model for exploring the complex integration and coordination of lipid metabolism, and corresponding metabolic signaling pathways, throughout the organism. For example: How does a major energy usage tissue, such as the muscle, communicate energy requirements with tissues that primarily synthesize, store, and supply lipids to the rest of the organism, such as adipose tissue?
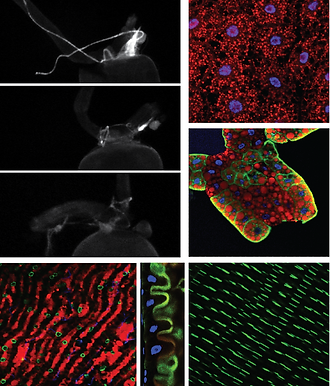




COORDINATION OF METABOLIC AND INNATE IMMUNE RESPONSES
for example: How and why do innate immune signaling pathways direct cellular metabolism?
How does diet impact innate immunity?
Do energetic trade-offs define innate immune signaling and host-pathogen responses?
How do uniques aspects of innate immune signaling impact tissue resilience?
Metabolic and innate immune responses, two primitive systems critical for the long-term homeostasis of multi-cellular organisms, have evolved to promote cooperative, adaptive responses against diverse environmental challenges. More directly, metabolic signaling pathways/transcription factors can shape innate immune responses through the regulation of innate immune gene expression, and innate immune responses (either cell-autonomously or through systemic inflammation) can alter metabolic signaling pathway activity as well as regulate metabolic gene expression.
We are currently using the fruit fly as model system in order to uncover the ancestral mechanisms that underlie the integration of metabolic and innate immune responses. Drosophila provide unique features, such as integrated organ systems, simplistic microbiota composition, and well-characterized bacterial enteropathogens, that can be leveraged to establish an innate immune-metabolic signaling framework. For example, the fly has many integrated organs (such as the fat body, a tissue most similar to mammalian adipose) that combine various nutrient and pathogen sensing (innate immune) systems in a single tissue, ie. before these systems evolved into more complex organ types in mammals. The lab is also interested in exploiting unique features of the Drosophila model to understand how distinct innate immune responses, or immuno-metabolic responses, influence epithelial tissue resilience and plasticity in response to stress.





MERGING MODELS TO STUDY DISEASE MECHANISMS
The Karpac lab is also coupling expertise in invertebrate and mammalian models to shed light on novel mechanism-based biomarkers for multifactorial (and complex) diseases. We are currently leveraging the unique experimental advantages of Drosophila, combined with collaborators and strategic approaches in mammalian and human models, to explore disease mechanisms. As a current example of successful integration and utilization of multiple models, we are merging in vivo Drosophila models, murine models, and ex vivo human models to explore the role of membrane-targeted dietary bio-actives in cancer signaling and tissue function. In collaboration with other labs, the Karpac lab is utilizing the Drosophila midgut (a tissue that is amendable to high-resolution microscopy) to explore the role of dietary bio-actives (such as n-3 PUFA and cholesterol dietary lipids) in shaping Ras and Wnt receptor nanoclustering at the plasma membrane in vivo - thus informing on the ability of these lipids to attenuate oncogenic signaling in murine and human models.






FUNDING SOURCES
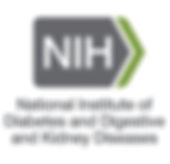
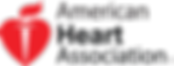

